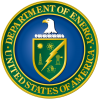
J. Mathias Weber, JILA and Department of Chemistry, Univ. of Colorado Boulder (PI)
Joel D. Eaves, Department of Chemistry, University of Colorado Boulder (Co-Investigator)
The proposed research will employ a combined experimental and theoretical approach to quantify interactions between water molecules and aromatic hydrocarbons (PAHs) at an unprecedented level of detail. Molecular clusters are excellent systems for theoretical and computational work, since they are sufficiently small for high-accuracy electronic structure calculations. At the same time, the precise control over experimental conditions in cryogenic cluster ion experiments, both through mass selection and cooling, dramatically reduces systematic uncertainties compared to analogous condensed phase work. Crucially, quantum nuclear effects that arise from non-classical, quantum behavior of protons in water often display modest effects in bulk liquid at room temperature. These effects are expected to be more pronounced in small water-PAH clusters, particularly at low temperature. Thus, the proposed systems will allow us to explore several different treatments for including quantum nuclear and many-body effects.
PAHs are valuable model systems for both neutral and charged graphene and their chemical derivatives. The water-graphene interaction is of paramount importance in materials chemistry. Theoretical predictions and careful experiments have shown that the graphene wetting characteristics are highly sensitive to small changes in the chemistry on the graphene surface. For example, application of small voltages applied to nanoporous graphene are predicted to increase water throughput in desalination applications, and the predicted water transport through such systems strongly depends on the details of the model for water-carbon interaction used. This implies that applications ranging from battery technology to electrocatalysis depend on interfacial phenomena that contemporary theoretical models only poorly describe. Experimentally probing the molecular level details of the interaction of single-layer graphene with water is also rather challenging, due to pitfalls in the preparation of clean single-layer graphene films and the size and shape heterogeneity of graphenic nanostructures synthesized in typical chemical preparations.
We will study the interaction of water molecules with charged PAHs in mass selected cluster ions of the form PAH+/-ยท(H2O)n, using cryogenic infrared photodissociation spectroscopy, and compare the experimental results with theoretical predictions. From an experimental point of view, using mass-selected PAH ions allows us to precisely control the size and shape of the graphenic system, as well as the number of water molecules interacting with it. This strategy leads to exquisitely well-controlled experimental conditions, because it removes fluctuations in the solvent environment and lets us study solvent interactions one molecule at a time. While this general strategy has been applied to many molecular systems, only recent advances in cryogenic ion spectroscopy allow us now to systematically investigate hydrated PAH cluster ions, particularly for larger PAHs and beyond ca. 5 water molecules.
We will parameterize the many-body intermolecular potential, and test both centroid and ring-polymer molecular dynamics approximations to relevant quantum time correlation functions associated with transitions between stable structures of the clusters and IR spectroscopy. The many-body potentials will be released as part of publicly available software package, maintained by Sandia National Labs, for molecular dynamics simulations.