Optical Atomic Clock | Pulse-timing Synchronization and Distribution | Photo-Association of Ultracold Sr Molecules
Our group explores many facets of ultracold strontium (Sr), emphasizing precision measurement and quantum-coherent manipulation and control of atoms and molecules. The group has achieved exquisite technical control via precision stabilization of lasers as well as atomic and molecular systems. Early on, the ultracold strontium group focused on precision measurements of Sr electronic transitions, which occur at optical frequencies, to explore the possibility of developing an optical atomic clock. In the early stages, the group focused on the development and understanding of laser-based two-stage Doppler cooling, the most straightforward method for cooling alkaline earth atoms to temperatures where precision frequency measurements are possible. The group performed systematic tests of laser cooling and trapping dynamics on the bosonic isotope 88Sr. A combination of strong and weak transitions enabled cooling of a large number of atoms to ultralow temperatures of 200 nK. The group also investigated direct sub-Doppler cooling of the fermionic isotope 87Sr using nuclear spin states. These cooling experiments led to the realization of absolute optical frequency standards based on ultracold 88Sr and 87Sr atoms in 2005 and 2006.
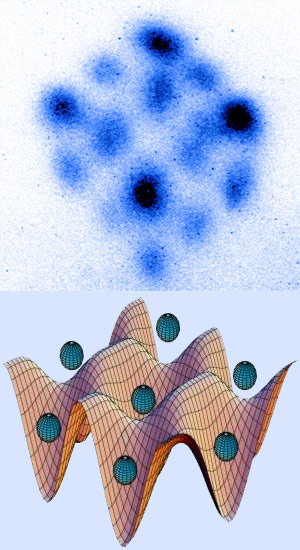
TOP: Image of momentum packets of laser-cooled 88Sr atoms
flying in formation away from the center of an atom cloud.
BOTTOM: Quantum mechanical view of same process.
The 88Sr experiments produced an interesting set of cooling dynamics that could be described by full quantum theory. The most surprising discovery came during an experiment employing six intersecting red laser beams in a magnetic field. When the laser frequency was tuned above the atomic resonance, the cold atom cloud divided into discrete momentum packets resembling lattice points on a face-centered cube. The packets were imaged flying in (cube-shaped) formation away from the center of the cloud. The group determined that the packets were created as they stopped absorbing laser photons (from different directions) and separated from the atom cloud. While this behavior should be universal for all laser-cooled atoms, the unique properties of Sr atoms made the first experimental observation of it possible in an ordinary-sized apparatus.
Once cooling processes were well understood, Ye group researchers turned their attention to investigations of electronic transitions in 88Sr and 87Sr, in anticipation of developing a neutral Sr-based optical atomic clock. Their initial goal was to perform precision measurements of Sr and understand its sensitivity to environmental parameters. This effort required the development of exquisitely sensitive precision measurement techniques. These techniques played a key role in developing an optical atomic clock. They also led to ever-more precise laser stabilization and increasing technical control of atomic and molecular systems. The latter accomplishments recently made it possible to undertake sophisticated experiments to manipulate and control cold atoms and molecules.
Our group's many technical achievements have created a bright future in ultracold strontium research. Current projects include the (1) precision enhancement of the 87Sr and 88Sr optical atomic clocks and (2) studies of cold molecules formed via photoassociation of ultracold 88Sr atoms. The group recently proposed a new precision test of the time-dependence of the proton/electron mass ratio in ultracold Sr atoms confined in an optical lattice. In the not-too-distant future, strontium may be explored as a candidate for quantum information processing because of the long coherence times observed between precision lasers and ultracold Sr atoms in an optical lattice. Ultracold Sr atoms confined in a lattice may also soon be used in direct experimental quantum-mechanical simulations of processes that are too challenging to solve with modern supercomputers. A quantum nondemolition measurement of the collective atomic pseudo-spin will lead to spin squeezing of the whole atomic system, further advancing the stability and accuracy of the Sr optical clock.
Optical Atomic Clock
Our group began its optical atomic clock work with investigations of transitions in neutral Sr atoms. The first experimental clock was built with 88Sr atoms, an abundant and stable natural bosonic isotope whose atomic properties are easy to understand because it lacks nuclear spin. The clock was created by using a cold 88Sr-based optical frequency standard to stabilize the repetition rate (ƒr) of a femtosecond optical frequency comb. It required strict knowledge of the frequency comb offset (ƒ0) due to pulse-to-pulse phase shifts. This arrangement made it possible to transfer precise and accurate optical phase information from the clock transition to the microwave domain, where it could be visualized and recorded electronically.
In 2005, the lab performed a precise and accurate measurement of 88Sr's naturally occurring clock transition (1S0→3P1). While the study provided useful information on the ultracold collision process between Sr atoms, the clock transition (7.3 kHz) was too wide to be a competitive candidate for a next-generation optical clock. The next step was to work with JILA theorist Chris Greene and his group to discover a better way of making an optical atomic clock based on a more stable transition. The collaboration succeeded with a design that uses two lasers and three atomic levels to create a unique energy-level transition that otherwise would not occur in nature. The new transition, which is due to quantum interference, is very narrow and highly stable. However, because it is not a natural transition, any clock based on it would require extensive and careful checks of possible errors introduced by the man-made transition scheme.
For this reason, our group decided to shift its short-term focus to the fermionic isotope 87Sr for lattice-based optical atomic clock development. Like 88Sr, 87Sr is a stable, neutral atom well suited for optical lattice trapping and amenable to laser cooling. However, it has a natural ultranarrow (~1 mHz) 1S0→3P0 clock transition, which the group was able to interrogate with stable diode lasers locked to an octave-spanning femtosecond frequency comb. The group made important improvements to this clock, including (1) a customized probe laser that is highly resistant to "noise" caused by vibration and gravity and (2) a refined optical lattice. First, the optical lattice (a single line of 100 pancake-shaped wells) was loaded by slowing the atoms with blue laser light and then using red laser light to further cool the atoms until they could be captured. Next, the atoms were precisely manipulated inside the lattice to prepare them in a suitable quantum state for clock operation. Finally, the influence of the lattice on the atomic transition frequency was systematically and precisely measured. The atoms' ticks (430 trillion per second) were probed with very stable red laser light at slightly different frequencies until the exact frequency at which the atoms absorb best was detected.
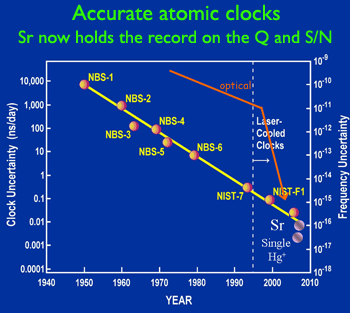
Recently, the new clock's absolute frequency transition was accurately measured to be 429 228 004 229 870.0(1.1) Hz. The group also used ultrahigh resolution spectroscopy to characterize the overall systematic uncertainty of the 87Sr clock's transition frequency as 9 × 10-16. This level is similar to the cesium fountain atomic clock, the nation's primary time-and-frequency standard. Recent work in the group has shown that the 87Sr lattice clock can reach an accuracy level comparable to Cs-fountain technology.
Our group has a strong collaboration with NIST-Boulder's Time-and-Frequency Division, where optical atomic clocks based on neutral Ca, Yb, and single Hg+ and Al+ ions are under development. The collaboration is helping local researchers rapidly improve their optical atomic clocks — to the point now where most of the optical atomic clocks perform so well in tests against the Cs fountain clock, they must now be tested against one another to make the most accurate and precise measurements. The Ye lab is currently testing its 87Sr lattice clock against NIST's Ca clock. Preliminary results suggest the 87Sr clock is more accurate and precise than the Cs fountain clock. The current test could be enhanced with a head-to-head comparison of the 87Sr lattice clock to the single Hg+ ion clock and its world record systematic uncertainty of 2-3 × 10-17. To further improve its optical atomic clock in the future, the Ye lab is investigating spin squeezing and quantum feedback control for improving the accuracy and precision of the 87Sr lattice clock.
Pulse-timing Synchronization and Distribution
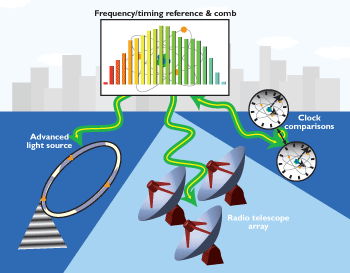
New technologies will rely on noise reduction strategies developed
in our lab for fiber network transfers of precision measurements
based on optical frequency combs.
The Ye lab participates in the optical clock comparisons by transmitting their clock signals back and forth across a 3.5-km fiber-optic link between JILA and NIST. The group began investigating the remote transfer of ultrastable frequency signals nearly a decade ago in anticipation that rapid development of optical atomic clocks might render GPS-based signal transfer inadequate. The new signal transfer system is already realizing its promise. At NIST, an optical frequency comb serves as an intermediate clock gear to facilitate comparison of the 87Sr lattice clock with other optical atomic clocks at NIST. This arrangement precisely and accurately transfers clock signals, thanks to recent work by the our group on coherent optical phase transfer over fiber networks. The group relied on a method for detecting and removing phase noise in a fiber link — a necessity since the local fiber-optic link is buried under Boulder city streets, where it is subject to traffic noise and temperature variations. To solve these problems, we heterodyne a signal that has made a round trip through a double-pass actuator and the fiber link against a reference. They then feed information about fiber-link noise contained in the heterodyne beat back into the actuator, which cancels the noise in the clock signal. Over time, the researchers have improved the stability of signal transmission over the fiber link by a factor of a hundred, ensuring that time can now travel even more accurately than the new 87Sr lattice clock can measure it.
Photo-Association of Ultracold Sr Molecules
Using precisely tuned laser light, our group has been able to use optical Feshbach resonances to induce a gas of ultracold 88Sr atoms to form stable Sr2 molecules. Light-catalyzed molecule formation is important because 88Sr atoms do not have magnetic Feshbach resonances, which promote the formation of other ultracold molecules such as 87Rb2. Photoassociation is a good way to create a large number of ultracold Sr2 molecules that are all in the same quantum mechanical state. And, understanding how these molecules form is yielding insight into the quantum mechanical behavior of the atoms themselves.
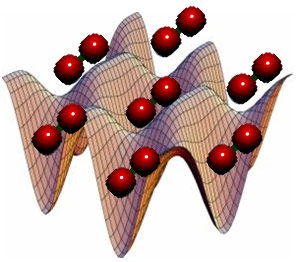
Ultracold 88Sr molecules in a lattice.
To make the molecules, researchers confine a cloud of a hundred thousand ultracold (2µK) 88Sr atoms in a one-dimensional "magic" wavelength optical lattice, i.e., a standing wave of far infrared-wavelength light. The lattice traps two-dimensional "pancakes" of 88Sr atoms at the antinodes of the standing wave. The lattice's 914 nm wavelength is magic because it has no net effect on transitions under investigation in the 88Sr atoms or Sr2 molecules, i.e., the ac Stark shifts of the two states of interest are equal. The magic wavelength lattice allows long interrogation times of the atoms, while simultaneously providing an environment that is free of perturbations. Atoms trapped in the lattice can be probed free of recoil or Doppler effects, making it possible to perform high-precision spectroscopy with high accuracy.
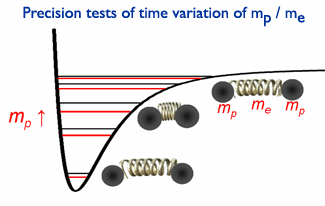
In the next year or so, we plan to make precise
measurements of the proton/electron mass ratio.
Trapped 88Sr atoms can be induced to form excited Sr2 molecules with a weak red laser at 689 nm. The narrow
transition (1S0→3P1) stimulated by this laser makes it possible to detect nine
excited molecular states with high resolution. It also favors the decay of the excited molecules into tightly bound and stable
ground-state molecules. The ground-state Sr2 molecules can then be used for precision measurements to help us understand
whether particular fundamental constants of nature have time dependence. For example, our group is planning to use Sr2
molecules to make precise measurements, separated by one year, of the proton/electron mass ratio (mp+/me-).
This measurement will be less complicated than measuring mp+/me- values at different epochs in the history of the Universe. However,
it will provide an important comparison to values measured astronomically. In addition, the Sr optical atomic clock itself can also
probe possible time variations of the fine structure constant, .